Paper: Ultra-wide-color-gamut LCDs with Vivid Color LED Technology
SID DisplayWeek 2017 Paper on Vivid Color Technology
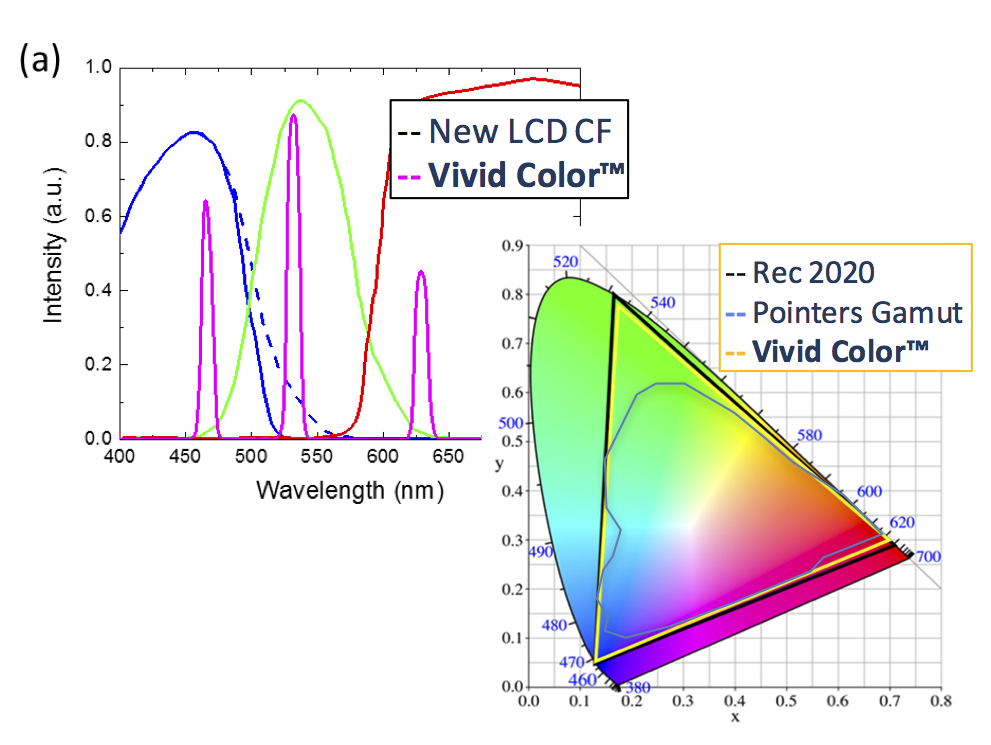
David Wyatt,* Haiwei Chen,** and Shin-Tson Wu**
*PixelDisplay Inc., San Jose, CA 95134, USA
**College of Optics and Photonics, University of Central Florida, Orlando, Florida 32816, USA
(c) 2016, PixelDisplay Inc. All Rights Reserved
The complete whitepaper can be found here : https://docsend.com/view/q7qpz96
Abstract
A Vivid Color™ LED with an ultra-narrow emission bandwidth (FWHM ~ 10 nm) has been developed. Using this LED backlight for a conventional TFT LCD, we can achieve 97.8% Rec. 2020 in CIE 1976 color space. The new LED has tunable emission characteristics, recycles light for improved power efficiency, and leverages existing LED and inorganic phosphor manufacturing processes for lower cost, and long lifetime. Widespread application in other displays, and lighting are foreseeable.
Keywords
Liquid-crystal display; Wide color gamut; Light-Emitting Diode; Augmented Reality; Holographic; Virtual Reality; LED; AR; VR; Micro-LED
1. Objective and Background
In the goal of enhancing realism, wider color gamut is of vital importance to all the display devices. For a non-emissive liquid crystal display (LCD), this could be achieved by optimizing the backlight technology, to generate more saturated red, green and blue (RGB) primary colors. In general, a light source with narrower FWHM (full width at half maximum) will lead to a wider color gamut. However, for the conventional backlight unit with YAG:Ce3+ phosphor-converted white light-emitting diode (WLED), its yellow spectrum is too broad (FWHM ~ 120 nm) so that the color gamut is relatively narrow [1]. To widen color gamut, two phosphor-converted WLED (aka "High-Gamut", "HG", "Narrow Phosphor LED") have also been developed [2-4]. Its green and red emission spectra are well separated, thus generating higher purity RGB primary colors. Nevertheless, the green band is still fairly broad (β-SiAlON:Eu2+ aka SIALON, FWHM ~55 nm) [5], also red emission (K2SiF6:Mn4+, aka KSF) is not deep enough [6]. Thus, its color gamut is limited to 70-80% Rec. 2020, depending on color filter.
Another promising light source is quantum dot (QD)[7-9].TheCd-based QDs exhibit a narrow FWHM (20 ~ 30 nm) and high luminescent quantum efficiency (> 95%)[8, 9].With commercially available color filters, 90% Rec. 2020 color gamut has been realized[10].However, cadmium is toxic. According to the Restriction of Hazardous Substances (RoHS) issued by the European Union [11], the maximum cadmium content should be limited to 100 ppm in any consumer electronic product. Therefore, heavy metal-free QDs, like InP QD, have become important alternatives[12, 13].But owning to the immature chemical synthesis process, InP QD’s quantum yield and stability are slightly inferior to those of Cd-based QDs. Importantly, the FWHM of InP QD is somewhat broader (> 40 nm),inhibiting the further enhancement of color gamut. Thus, the challenge remains: to find a light source with narrower FWHM, high efficiency, long lifetime, environmental friendly and excellent stability.In this paper, we report a new light source design: Vivid Color™, which can outperform the color-spectral purity of the aforementioned technologies. With an FWHM of 10 nm an exceedingly high color gamut (97.8% Rec. 2020 in CIE 1976) is achievable. By focusing on existing LED junction and phosphor design for stability and RoHS compliance and incorporating an energy recycling design, a near-term productizable solution for wider gamut color backlighting is achieved. We believe this Vivid Color™ LED will have widespread applications for future LCDs.
2. Results
The Vivid Color™ LED developed leverages advances in light recycling to optimize color purity, while enhancing energy-efficiency. An InGaN junction, with YAG phosphor, can be the core of the basic design, adding nitride, silicate and aluminate based-phosphors as desired to tailor the spectral output. It should be noted that all the materials in our new LEDs contain no heavy metals, and are compliant with RoHS regulation. For example stronger red emission using a Strontium Nitride-based phosphor provides long-lifetime stability, has abundant supply and Sr is biologically similar to Calcium.
Due to its unique design, our Vivid Color™ LED exhibits an ultra-narrow FWHM. Figure 1 shows an example of the measured emission spectrum of our new LED. The spectral results were recorded with an NIST calibrated CR-250 from Colorimetry Research. For comparison, we also include the conventional 1pc-WLED in Fig. 1.
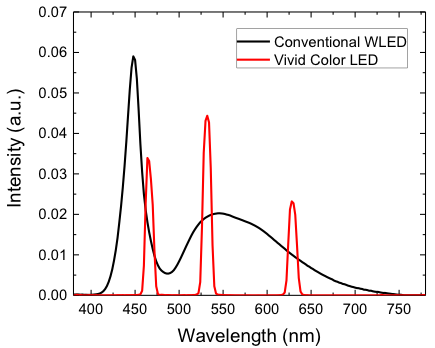
Figure 1. Measured emission spectra for conventional WLED and Vivid Color™ LED.
FromFig. 1, the peak wavelengths for three primary colors areλB = 467 nm, λG = 532 nm, and λR = 630 nm, which are consistent with the three primary colors of Rec. 2020 standard [14]. However, as will be discussed later, the optimal central wavelengths should be slightly deviated from the standard ones, especially for blue light. Fortunately, the emission peaks are tunable to different requirements. Presently, the FWHM is 10 nm for all three primary colors. This value is 2X smaller than that of the best QDs (~25 nm), thus the color gamut is widened. However in rendering smaller color gamut such as DCI-P3, larger FWHM primaries are advantageous for improved power-efficiency.To investigate the color performance using our Vivid Color™ LED, we chose a fringe field switching (FFS) LCD with negative dielectric anisotropy (Δε < 0) LC [15, 16] and a commercial color filter array as an example [Fig. 2(a)]. Such an LCD has been widely used in smartphones and pads. The wavelength dependent refractive indices of the employed LC are also considered in the simulation. The obtained results are summarized in Table 1. They are 86.3% Rec. 2020 in CIE 1931 and 75.4% Rec. 2020 in CIE 1976 color space. This is somewhat lower than our expectations. To fully understand the underlying physical mechanism, we plot the corresponding chromaticity coordinates in CIE 1931 and CIE 1976, as shown in Figs. 2(b) and 2(c). From these two figures, it is clearly seen that red coordinate almost overlaps with the standard, but the blue and green colors deviate from the references. Especially for blue color, its coordinate shifts noticeably from the desired position. This discrepancy results from the severe crosstalk between the blue and green color filters.
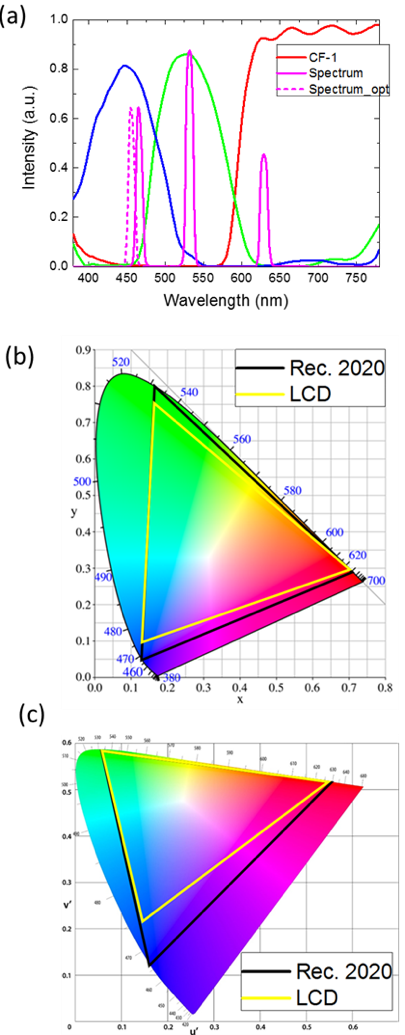
Figure 2. (a) Transmission spectrum of color filter CF-1, and emission spectrum of Vivid Color™ LED (dashed line is optimized emission spectrum); (b) Color gamut in CIE 1931 and (c) color gamut in CIE 1976.
Table 1. Calculated color gamut using CF-1 color filter.
Figure 3 depict the output spectra after LC layer and color filters for three primary colors. As expected, there is severe light leakage for each channel, especially for blue, where a relatively large bump leaks through the green color filter, which in turn deteriorates the final color purity. This explains why blue color coordinate shifts towards the green region in color space. Therefore, to enlarge color gamut, the optimal blue central wavelength should be slightly shorter, in order to compensate the unwanted green light leakage. As Fig. 2(a) depicts, we intentionally shift blue emission to 457 nm (dashed lines), and recalculate the color gamut. Results are included in Table 1. Expectedly, its color gamut is enhanced to 92.3% Rec. 2020 in CIE 1931, and 92.5% Rec. 2020 in CIE 1976.
Figure 3. Output spectra for blue, green and red primary colors, respectively.
Color filter is another important factor to determine an LCD’s color gamut. In Fig. 2(a), a high efficient color filter (CF-1) is employed, but it exhibits large crosstalk. Here, we choose another color filter (CF-2) with much narrower transmission bands, but its optical efficiency is compromised [Fig. 4(a)]. The obtained results are summarized in Table 2. They are 92.6% Rec. 2020 in CIE 1931 and 84.7% Rec. 2020 in CIE 1976 color space. Also, we plot their color coordinates in corresponding chromaticity diagrams, as shown in Figs. 4(b) and 4(c). This time, the deviation is much smaller, owing to the suppressed crosstalk [Fig. 5]. If we slightly adjust the central wavelengths as listed in Table 2, the resultant color gamut can reach 94.5% Rec. 2020 in CIE 1931 and 94.2% Rec. 2020 in CIE 1976.
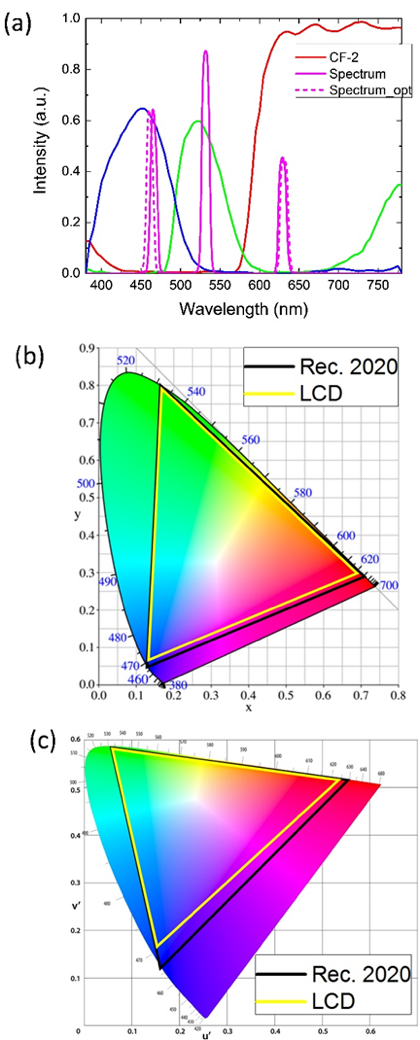
Figure 4. (a) Transmission spectrum of color filter CF-2, and emission spectrum of Vivid Color™ LED (dashed line is optimized emission spectrum); (b) Color gamut in CIE 1931 and (c) color gamut in CIE 1976 color space.
Next, we fine-tune the FWHM of our Vivid Color™ LED and investigate how it affects the final performance. For easy identification and fair comparison, we fix the central wavelength of three primary colors, i.e. λB = 462 nm, λG = 532 nm, and λR = 632 nm, and change their FWHM, as shown in Fig. 6. Here, CF-2 is employed. Results are summarized in Table 3.
When FWHM is increased from 10 nm to 15 nm, color gamut is decreased from 94.5% to 93.7% Rec. 2020. If we further increase the FWHM to 25 nm, color gamut is decreased to 90.6% Rec. 2020.
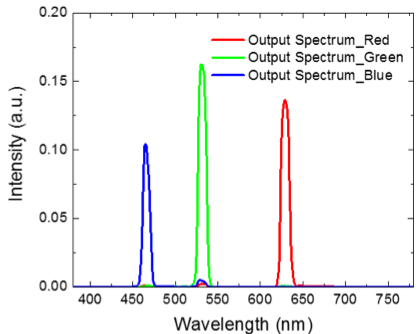
Figure 5. Output spectra for blue, green and red primary colors, respectively.
This is comparable to that of Cd-based QDs. When FWHM is 45 nm (InP QD), its color gamut is reduced to 81.9% Rec. 2020. This comparison is based on CIE 1931. For CIE 1976, it shows similar trend, but with different values, as shown in Table 3.
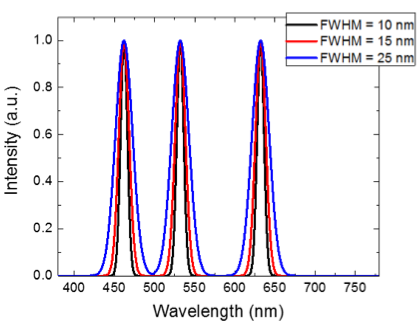
Figure 6. Vivid Color™ LED with different FWHMs. (λB = 462 nm, λG = 532 nm, and λR = 632 nm)
... (truncated) ...The complete whitepaper can be found here : https://docsend.com/view/q7qpz96